Molecular Operating Environment Installation Wizard
- Molecular Operating Environment Download
- Molecular Operating Environment Installation Wizard101
- Operating Environment Vs Operating System
Keywords: Integrated Protein Engineering Applications; Residue scanning to identify critical residues for affinity; Search for optimal mutations to modulate. Apr 21, 2015 - CII259–273 1 (Fig. 1) – a glycopeptide fragment derived from type II collagen – is presented to CD4+ T-cells by Aq MHC molecules in CIA. Molecular Operating Environment (MOE), 2012.10; Chemical Computing Group Inc., 1010 Sherbrooke St. West, Suite #910, Montreal, QC, Canada, H3A 2R7, 2012.
Received 26th February 2015, Accepted 21st April 2015 First published on 21st April 2015 Class II major histocompatibility complex (MHC) proteins are involved in initiation of immune responses to foreign antigens via presentation of peptides to receptors of CD4 + T-cells. An analogous presentation of self-peptides may lead to autoimmune diseases, such as rheumatoid arthritis (RA). The glycopeptide fragment CII259–273, derived from type II collagen, is presented by A q MHCII molecules in the mouse and has a key role in development of collagen induced arthritis (CIA), a validated model for RA.


We have introduced hydroxyethylene amide bond isosteres at the Ala 261–Gly 262 position of CII259–273. Biological evaluation showed that A q binding and T cell recognition were dramatically reduced for the modified glycopeptides, although static models predicted similar binding modes as the native type II collagen fragment. Molecular dynamics (MD) simulations demonstrated that introduction of the hydroxyethylene isosteres disturbed the entire hydrogen bond network between the glycopeptides and A q. As a consequence the hydroxyethylene isosteric glycopeptides were prone to dissociation from A q and unfolding of the β 1-helix.
Thus, the isostere induced adjustment of the hydrogen bond network altered the structure and dynamics of A q/glycopeptide complexes leading to the loss of A q affinity and subsequent T cell response. Introduction Rheumatoid arthritis (RA) is an autoimmune disease that mainly affects peripheral joints by causing inflammation that destroys cartilage and bone. The cause of the disease is not yet known, but a genetic link has been found to the expression of the class II major histocompatibility complex (MHC) proteins HLA-DR1 and -DR4. In collagen-induced arthritis (CIA), a validated mouse model for studies of RA, development of disease is linked to expression of A q MHC II molecules. Class II MHC proteins are found in antigen presenting cells (APC) where they are associated with peptides derived from endocytosed protein antigens. The complexes are then transported to the surface of the APC and presented to T-cell receptors (TCR) on circulating CD4 + T-cells, leading to initiation of an immune response towards the original antigen.
CII259–273 1 – a glycopeptide fragment derived from type II collagen – is presented to CD4 + T-cells by A q MHC molecules in CIA. Glycopeptide 1 has also been proven to work as a vaccine that effectively prevents development of disease in CIA when administered as a complex with A q. The complex also reduces disease progression and severity in a mouse model of chronic relapsing arthritis. In humans, glycopeptide 1 binds to DR4 and the complex is recognized by T-cells isolated from patients suffering from severe RA, suggesting 1 to have a central role in RA just as in CIA.
The minimal epitope in 1 required for binding to A q, and stimulation of CII restricted T cell hybridomas obtained in CIA, consists of amino acid residues Ile 260–Gln 267. Antigenic peptides bind between the α 1- and β 1-helices of class II MHC proteins through a hydrogen bond network and by anchoring of sidechains in pockets in the binding groove.
Synthesis and biological evaluation of a comprehensive panel of synthetic analogues of 1 has revealed Ile 260 and Phe 263 to be crucial anchor residues that bind in hydrophobic pockets of A q. Moreover, the galactosylated hydroxylysine at position 264 protrudes out of the binding site and is recognized by the TCR with exquisite specificity. Scheme 1 Reagents and conditions: (a) LiAlH(O- tBu) 3, EtOH, −78 °C, 75%; (b) ( S)-alpine-hydride, THF, −78 °C, 84%; (c) (i) TFA, CH 2Cl 2, rt; (ii) FmocOSu, Na 2CO 3, dioxane, H 2O, 0 °C → rt, 86% for 8 from 6, 48% for 9 from 7 (after separation of the diastereomers by chiral chromatography); (d) (i) tBuSH, AlMe 3, CH 2Cl 2, 0 °C; (ii) TBDMSCl, imidazole, DMF, rt, 55% for 10 from 8 and 85% for 12 from 9; (e) LiOH, H 2O 2, THF, 0 °C → rt, 75% for 11 from 10 and 82% for 13 from 12. The isostere building blocks 11 and 13 were incorporated into the CII259–273 glycopeptide sequence using Fmoc-based SPPS under standard conditions. The isosteric glycopeptides were cleaved from the solid support, deacetylated, and purified by reversed-phase HPLC to give the trifluoroacetate salts of 2 and 3 with overall yields of 18 and 28%, respectively, and in 98% purity.
All glycopeptides were homogeneous, according to analytical reversed-phase HPLC and their structures were confirmed by 1H NMR spectroscopy and MALDI-TOF mass spectrometry. Binding to the A q protein and recognition by T-cell hybridomas The ability of the hydroxyethylene-modified glycopeptides 2 and 3 to bind to the A q protein compared to 1 was assessed in a competitive ELISA binding assay. Significant optimisation was undertaken to obtain a satisfactory signal to noise ratio in the ELISA, which led to the use of rather high concentrations of the biotinylated class II-associated invariant chain peptide (CLIP) tracer. As the CLIP binds with high affinity to A q higher concentrations of weaker inhibitors, such as native 1, had to be employed in the ELISA. No binding could be detected for 2 and 3 even at the highest concentration of 1 mM where 1 completely competed out of the biotinylated CLIP tracer. However, it is likely that a weak binding of 2 and 3 to A q would not be detected under the conditions that had to be employed in the ELISA. Replacement of the Ala 261–Gly 262 amide bond with the two different hydroxyethylene isosteres thus had severe effects on the glycopeptide abilities to bind to A q.
These results underscore our previous results that this particular amide bond is highly sensitive to modifications. At this position the corresponding ketomethylene-isostere 4 is the only modified glycopeptide so far that has been shown to bind equally well to A q as 1. 3 Binding of native glycopeptide 1 and hydroxyethylene isosteres 2 and 3 to A q. In the competitive binding assay, increasing concentrations of 1, 2, or 3 were incubated with A q protein and a fixed concentration of a biotinylated CLIP peptide. After the incubation, the A q-bound CLIP peptide was detected in a time-resolved fluoroimmunoassay using europium-labeled streptavidin. The points represent the average of duplicates and error bars are set to ±one standard deviation.
The T-cell response was investigated in a cell-based assay, where IL-2 secretion of A q restricted T-cell hybridoma lines was measured after incubation with antigen-presenting spleen cells expressing A q and increasing concentrations of the glycopeptides 1, 2 or 3. Four A q-restricted T-cell hybridomas that differed in their TCR specificity for the hydroxyl groups of the galactose moiety were investigated for their ability to recognize the glycopeptides. Despite the fact that no binding of 2 and 3 to A q was detected by the competition assay, both were recognized and stimulated IL-2 secretion by HCQ.3 and HM1R.2, while the other two hybridomas HCQ.10 and 22a1-7e did not give any response.
Molecular Operating Environment Download
The HCQ.3 and HM1R.2 responses of 2 and 3 were significantly weaker compared to the responses elicited by both the non-modified 1 and ketomethylene-isosteric glycopeptide 4; the latter of which elicited intermediate to strong responses from three of the four hybridomas. This suggests that 2 and 3 do in fact bind to A q and were presented to the T-cell receptor in the functional assay, although too weakly to be detected in the binding assay. 4 Recognition of glycopeptides 1–3 by the A q-restricted T-cell hybridomas HCQ.3, HCQ.10, HM1R.2, and 22a1-7e after incubation with antigen-presenting spleen cells expressing A q and increasing concentrations of 1–3. Recognition of the peptide/MHC complex by the T-cell hybridomas results in secretion of IL-2, which was quantified by a sandwich ELISA using the DELFIA system. That HCQ.10 and 22a1-7e were more sensitive towards the introduced modifications in the backbone compared to HCQ.3 and HMIR.2 has also been seen in previous studies. Analysis of glycopeptide/A q-complexes by MD simulations Glycopeptides 1–4 were modelled into A q to understand if the structures of the complexes correlated with the binding affinity for A q and with the subsequent T cell responses.
We found that the modified glycopeptides 2–4 displayed a binding mode almost identical to that of the native glycopeptide 1 in their respective complexes. Similar interactions were retained for all four glycopeptides although the position of the hydrogen bond acceptor had been altered for the hydroxylethylene-isosteric glycopeptides 2 and 3 compared to the carbonyl oxygen in 1 and 4. As the static structures were unable to explain the observed differences in A q binding and T cell responses the dynamics of the isostere modified glycopeptide/A q-complexes and the native system were studied over time using MD simulations. Repeated 60 ns MD simulations at different initial velocities were conducted for 1 (two times) and the modified glycopeptides 2–4 (three times) in a complex with A q, using the static models as starting structures. The repeated runs will hereafter be referred to as (I), (II) and (III) and can be seen as samples from the complete conformational landscape of the systems. In general, the simulations reached equilibrium after 40 ns according to the root-mean-square deviation (RMSD) values (see the ESI for RMSD plots).
The analyses of the MD simulations were then focused on the binding mode and flexibility of the glycopeptides, and the hydrogen bonding network between the modified glycopeptides and A q over the last 20 ns of the repeated runs. The system of 1/A q served as a reference to which the modified glycopeptides 2–4 have been compared. Flexibility of glycopeptide binding to A q During the simulations, glycopeptide 1 was bound in the groove-shaped binding site of A q. The N-terminus remained firmly anchored in the binding groove, mediated by a network of intermolecular hydrogen bonds and by positioning of the anchor residues Ile 260 and Phe 263 in the P1 and P4 pockets, respectively.
The C-terminus on the other hand proved to be much more flexible and did not adopt a stable binding mode during the course of the simulations. This is consistent with previous MD simulations of the system, as well as the fact that the minimal epitope required for binding to A q consists of the N-terminal octapeptide Ile 260–Gln 267. 5 Snapshots from the MD-simulations comparing the mobility of the A q/glycopeptide complexes of 1–3. (A) Superposition of frames extracted over the last 20 ns of the MD simulations, comparing native 1 (I) (in green, left) to 2 (I) (in orange, right). Glycopeptides 2 and 3 (data not shown) both display a higher mobility than the native glycopeptide 1.
In order to extract representative snapshots, frames have been randomly selected from each of the top 10 clusters for the minimal epitope (Ile 260–Gln 267) over the last 20 ns of the MD simulations. (B) Comparison of structures of glycopeptides 1–3 bound to A q. The representative snapshots have been randomly selected from the most highly populated cluster for the minimal epitope (Ile 260–Gln 267) over the last 20 ns of the MD simulations. In contrast to native 1, isosteric glycopeptides 2 and 3 both dissociate from the binding groove of A q, here illustrated by comparison of the structure of 1 (I) (green) to 2 (III) (in orange, left) and 3 (II) (in purple, right).
Vmix 20 full crack. The download above includes the latest version of vMix, vMix Social, vMix Title Designer and vMix Desktop Capture all in the one setup program. VMix is a Windows. VMix 17 Vmix 14 crack free download. 91 is a software to carry out the transmission through the biggest streaming sites such as Twitch TV, YouTube or Ustream. Vmix 14 Serial Crack With Keygen is a amazing with latest technology mixing software that utilizes the latest advances in your PC hardware with HD mixing.
A complete list with figures displaying superposed frames of the complexes from the last 20 ns of each MD simulations can be found in the ESI. See the Experimental section for details on the clustering.
The roman numbers I, II and III refer to the repeated MD simulations conducted for the same glycopeptide. The S- and R-hydroxyethylene-isosteric glycopeptides 2 and 3 displayed a different dynamic pattern compared to 1, with large variations between the repeated MD simulations ( and ESI). The S-hydroxyethylene-isostere 2 showed larger movements; the N-terminus of the glycopeptide was not as firmly anchored in the binding groove as for 1 and displayed a high degree of flexibility ( and the ESI).
In fact, the anchor residue Phe 263 and occasionally also Ile 260 were leaving their respective binding pockets. In addition, a clear unfolding was observed in the β 1-helix at the point of the modification of the peptide. The R-hydroxyethylene-isostere 3 also displayed larger flexibility; again both anchor residues were leaving their respective binding pockets and the β 1-helix was unfolded adjacent to the isostere in 3. The simulations of the ketomethylene isostere 4 in complex with A q displayed a similar dynamic behaviour as the native glycopeptide 1, including firmly anchored side chains of Phe 263 and Ile 260 and a stable β 1-helix. I, II and III represent the different MD simulations conducted for the same glycopeptide.
Strong hydrogen bonds have an occupancy above 40%. Weak hydrogen bonds have an occupancy above 10% and under 40%. The results of the repeated MD simulations are different scenarios or time periods. While some of the MD simulations for 2 and 3 displayed a behaviour indicative of a poor binder about to completely dissociate from A q other simulations indicated a plausible binder instead.
It should be noted that in previous MD simulations of the native glycopeptide 1, we have not observed a behaviour similar to 2 and 3, with the peptide dissociating from the binding groove, anchor residues leaving the P1 and P4 pockets, or unfolding of the β 1-helix. Hydrogen bond network An extensive hydrogen bond network anchored glycopeptide 1 into A q during the molecular dynamics simulations.
In total, 13 different hydrogen bonds were observed between the backbone of 1 and A q, and 7 of these had over 40% occupancy during the simulation ( and ). The majority of hydrogen bonds were formed between the N-terminal part of 1 (Gly 259–Gly 265) and A q, which was consistent with previous findings. In this sequence, 8 out of 13 intermolecular hydrogen bonds were present, of which 5 were considered strong bonds. In particular two hydrogen bonds showed a high presence during the MD simulations, Ala 261(a)–Asn 82(s) and Gln 267(a)–Trp 61(s). General procedure for solid-phase peptide synthesis. Glycopeptides 2 and 3 were synthesized in mechanically agitated reactors on a TentaGel-S-PHB-Thr( tBu)-Fmoc resin (40 μmol) using standard solid-phase methodology essentially as described elsewhere.
All couplings were performed in DMF. N α-Fmoc amino acids with standard side-chain protecting groups (4 equiv.) were activated with 1-hydroxybenzotriazole (HOBt, 6 equiv.) and 1,3-diisopropylcarbodiimide (DIC, 3.9 equiv.) and the couplings were monitored using bromophenol blue as the indicator. (5 R)- N α-(Fluoren-9-ylmethoxycarbonyl)- N ε-benzyloxycarbonyl-5- O-(2,3,4,6-tetra- O-acetyl-β- D-galactopyranosyl)-5-hydroxy- L-lysine (1.5 equiv.) and the isostere building blocks 11 and 13 (1.5 equiv.) were activated with 7-aza-1-hydroxybenzotriazole (HOAt, 3 equiv.) and DIC (1.5 equiv.) and coupled for 24 h. Fmoc deprotection after each coupling cycle was accomplished by treatment with 20% piperidine in DMF for 10 min.
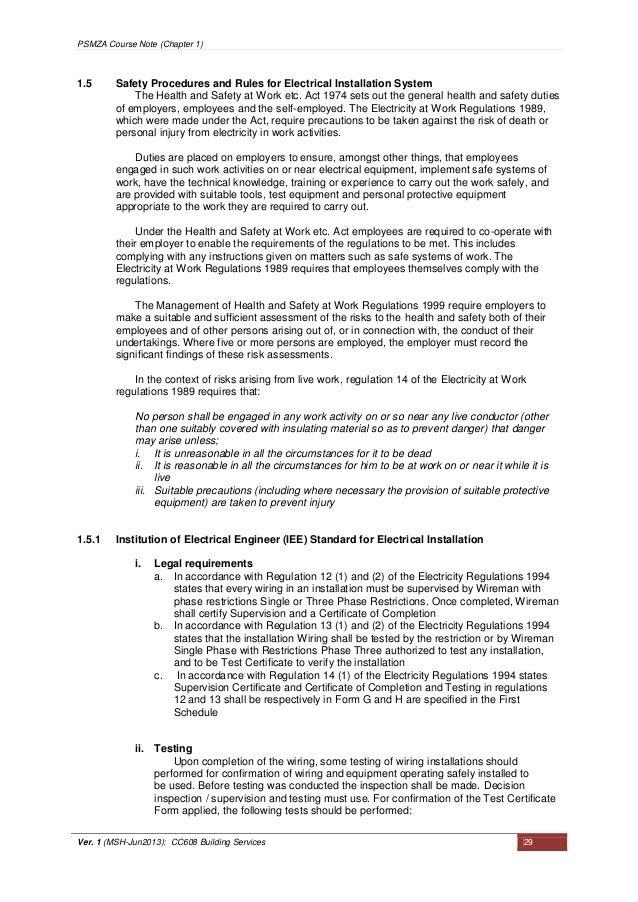
The glycopeptides were cleaved from the resin with trifluoroacetic acid/H 2O/thioanisole/ethanedithiol (35: 2: 2: 1, 40 mL) for 3 h at 40 °C with workup performed essentially as described elsewhere. Purification by reversed-phase HPLC was followed by deacetylation with NaOMe in MeOH (20 mM, 1 mL per mg peptide) for 2–3 h at rt (monitored by analytical reversed-phase HPLC). The mixture was neutralized by addition of AcOH and concentrated under reduced pressure, and the residue was finally purified using reversed-phase HPLC followed by lyophilization. Glycyl- L-isoleucyl- L-alanylψ(4S)-CHOHCH 2glycyl- L-phenylalanyl-(5R)-5-O-(β- D-galactopyranosyl)-5-hydroxy- L-lysylglycyl- Lglutam-1-yl- L-glutaminylglycyl- L-prolyl- L-lysylglycyl- L-glutam-1-yl- L-threonine ( 2).
Synthesis was performed with building block 13 according to the general procedure described above. This afforded the trifluoroacetate salt of 2 (12.4 mg, 18% yield based on the amount of resin used) as a white amorphous solid after lyophilization. MS (MALDI-TOF) calcd 1654.82 for M + H +, found 1654.82.
1H NMR data are given in. Residue NH H α H β H γ Others Measured at 500 MHz and 298 K in water containing 10% D 2O with H 2O ( δ H 4.76 ppm) as an internal standard. Degeneracy has been assumed. Chemical shifts for the galactose moiety: δ 4.43 (H1), 3.91 (H4), 3.76 (H6), 3.68 (H5), 3.64 (H3), and 3.52 (H2). Residue NH H α H β H γ Others Measured at 500 MHz and 298 K in water containing 10% D 2O with H 2O ( δ H 4.76 ppm) as an internal standard. Degeneracy has been assumed. Chemical shifts for the galactose moiety: δ 4.43 (H1), 3.92 (H4), 3.76 (H6), 3.68 (H5), 3.64 (H3), and 3.52 (H2).
Parameter preparation GalHyl 264. Partial charges for the non-standard residue GalHyl 264 were computed using the R.E.D. Server/R.E.D IV Seven conformations of the ACE–GalHyl 264–NME capped amino acid were used, extracted from a conformational search using the OPLS 2005 force field and a dielectric constant of 80 as implemented in the Macro model within Maestro. The partial charges were thereafter fitted to reproduce molecular electrostatic potential (MEP). The intra-molecular charge constraints for ACE and NME were set to zero during the charge fitting step and are thereafter removed from the molecule to give a force field library for the central fragment only. Using AnteR.E.D. 2.0, P2N files were generated, thereafter manually modified to correct the atom order and connectivity, and finally joined together into one single file.
The R.E.D IV server with the RESP-A1 (HF/6-31G.) charge model was thereafter applied. The GLYCAM force field atom types were assigned for the carbohydrate part and AMBER force field atom types for the amino acid part. Amide bond isosteres. The coordinates for the three amide bond isosteres were taken from the prepared complexes as described above.
Molecular Operating Environment Installation Wizard101
The partial charges for the amide bond isosteres were thereafter derived following a similar procedure as described above for GalHyl 264 using the R.E.D server and the RESP-A1 (HF/6-31G.) charge model. The central fragment procedure was applied using the capped amide bond isostere fragment with an acetylated N-terminus (ACE) and a methylated C-terminus (NME) using one conformation for each amide bond isostere.
Operating Environment Vs Operating System
Books.google.com.ua - As the pharmaceutical industry continues to advance, new techniques in drug design are emerging. In order to deliver optimum care to patients, the development of innovative pharmacological techniques has become a widely studied topic.Applied Case Studies and Solutions in Molecular Docking-Based Drug. Applied Case Studies and Solutions in Molecular Docking-Based Drug Design.